Molecule's carbon chain length affects oxygen's departure in key reaction for building bio-fuels
In a maze of blindingly complex reactions that snap oxygen atoms off cellulose or other bio-sources to create energy-dense fuel, the starting molecule's size has a curious effect. If the oxygen-rich molecule is too short to comfortably stretch to a catalyst's active site, oxygen atoms are split from its hydrocarbon chain instead of staying together as happens when the molecule can reach across, according to scientists at Pacific Northwest National Laboratory (PNNL) and Baylor University. The team uncovered this steric effect by comparing two cellulose stand-ins that each have two oxygens or hydroxyl groups. Iterating between experimental and computational studies, they learned that the longer molecule keeps its last oxygen until the last step. The shorter one drops its oxygen atoms earlier as it struggles to fit on the catalyst's surface.
"It's safe to say that we didn't expect the chemistry to be this complex," said Dr. Roger Rousseau, a computational chemist at PNNL who worked on the study. "We've done a lot of research into alcohols, but extrapolating from one hydroxyl group to two was an order of magnitude more complex."
Why It Matters: Economic, environmental, and security concerns are tied to the global need for energy. World energy consumption is predicted to grow by 56 percent between 2010 and 2040, with almost 80 percent of that energy coming from fossil fuels. Replacing those fuels in industrial applications could reduce these concerns. However, transforming bio-feedstock into fuels means quickly and efficiently removing oxygen atoms. To do this, scientists need to understand how and why the atoms behave as they do. This study uncovers the hidden reactions using molecular stand-ins, known as diols, on a prototypical catalyst.
"It looks as if it should be simple; you pull the oxygen off the biomass and get hydrocarbons. The reality is that it is a pretty complex reaction with a lot of intermediate steps," said Dr. Zdenek Dohnálek, an experimental chemist at PNNL who led the research. "Our research -- generating the elementary steps in oxygen removal -- is contributing to an uncharted area."
Methods: To resolve the complexity of the reactions, the team compared the reaction of two diols on the prototypical oxide catalyst titanium dioxide. The diols were a longer 1,3-propylene glycol (HO(CH2)3OH) with a three-carbon backbone and the shorter ethylene glycol (HO(CH2)2OH) with just two carbon atoms.
"It took more than three years to compare and contrast the reactions," said Rousseau. "We'd come up with new ideas to explain what we were seeing. We'd measure. We'd do the calculations. And then, we'd do it all again until we knew what was happening," said Rousseau.
The measurements came from scanning tunneling microscopy (STM); the calculations, from complex density functional theory. Using STM and temperature-programmed desorption, the team determined which bonds were broken and which intermediates formed. "STM was critical to providing information," said Dohnálek. "In a sense, it was the only way we could disentangle what was happening -- imaging one molecule at a time."
The experimental team deposited each diol in a thin layer on titanium dioxide at low temperatures. The diol landed on the titanium rows of the catalyst with the molecule's oxygen atoms resting on the row of titanium atoms. The attached hydrogen in the hydroxyl group hopped on and off.
"This was as expected," said Dohnálek. "Then, it got surprisingly complex."
The team determined that the longer 1,3-propylene glycol reached out. The hydroxyl dropped into a nearby oxygen vacancy, a "hole" in the surface. The associated hydrogen broke off. "This was a standard acid base reaction that we have seen for alcohols," said Dohnálek.
The shorter ethylene glycol couldn't quite reach the oxygen vacancy. The hydroxyl group broke off from the hydrocarbon chain completely as the molecule struggled to reach the oxygen vacancy.
"This mechanism is different than what we typically see for alcohols," said Rousseau.
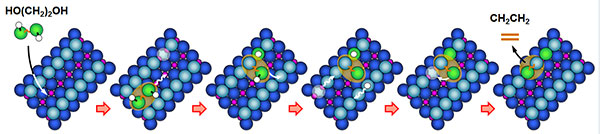
Ethylene glycol lands on titanium dioxide catalyst, with the oxygen atoms (green) resting on the row of titanium atoms. The attached hydrogen atoms (white) hop onto the nearby oxygen atoms (light blue). One of the molecule’s oxygen atoms falls into a nearby oxygen vacancy. At higher temperatures, the second oxygen atom also falls into another oxygen vacancy, which migrated across the surface. Heating leads to the cleavage of both oxygen atoms, leaving behind a hydrocarbon that comes off the surface. This sequence of steps, which are of interest to catalytic scientists, was described by the research team at PNNL and Baylor University. Copyright 2013. American Chemical Society
When the temperature was raised above ~400 K, they saw a new stable intermediate centered on the bridging oxygen row. This intermediate was a new dioxo species. Further heating led to the homolytic cleavage of the other oxygen, and the hydrocarbon then left the surface when the temperature was raised above ~500 K.
"Again this process was very different from the one we saw for alcohols as it proceeded by a hemolytic rather than heterolytic bond breaking and was a nonadiabatic," said Rousseau.
"The reactions are wonderfully complex and fun to study," said Dohnálek.
What's Next? The team will be diving into further complexity when they apply what they've found here to tungsten trioxide catalysts and other catalytic materials.
No comments:
Post a Comment